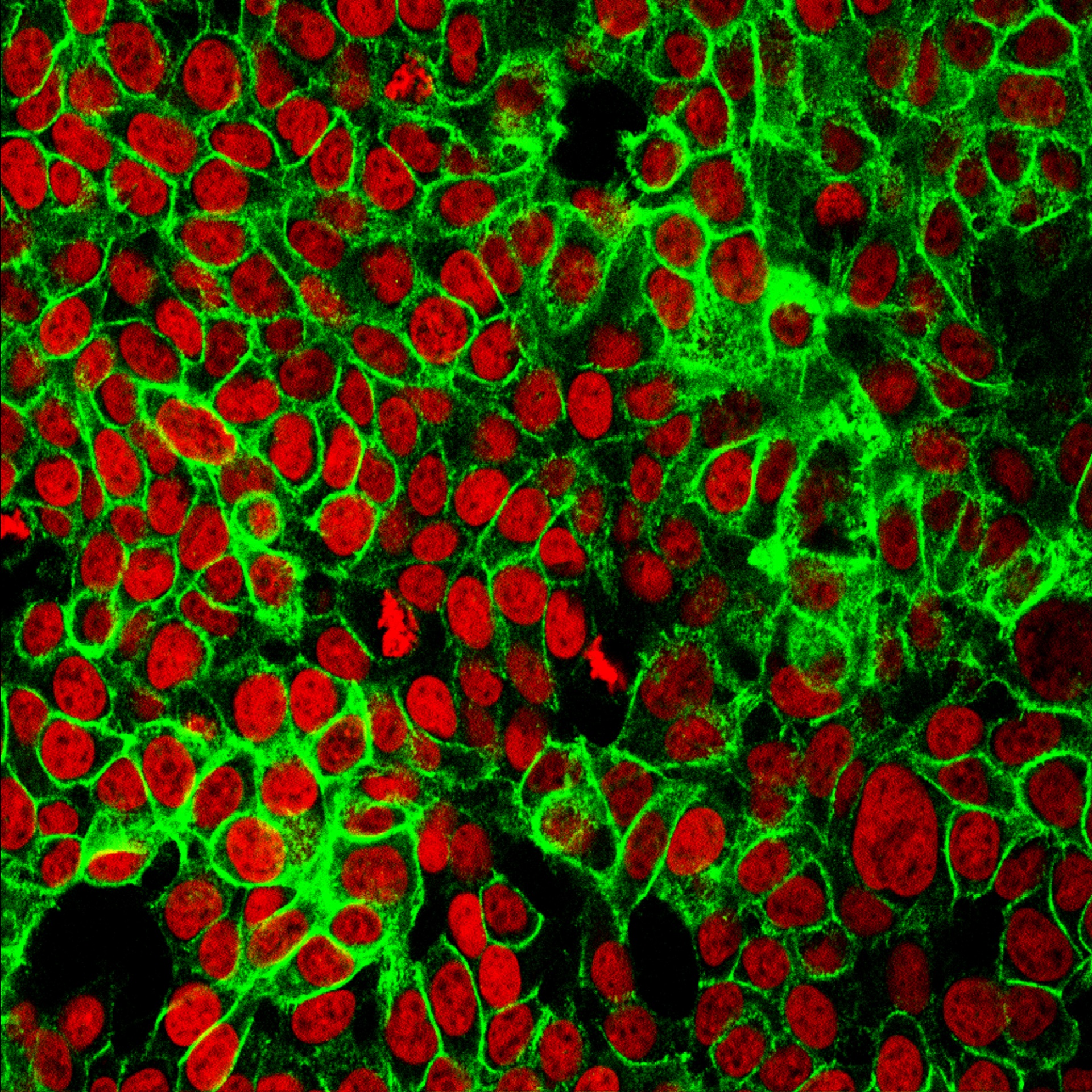
Structural study of cotranscriptional spliceosomes stalled by antitumor compounds
Project background
Splicing is a critical step in gene expression, responsible for removing non-coding introns from pre-mRNA transcripts. This process can occur in alternative ways, enabling the production of multiple mRNA variants from a single gene. Splicing involves two chemical reactions that utilize reactive groups from distinct regions of the pre-mRNA: the branch site adenosine and the 5' and 3' splice sites. Despite the seemingly straightforward chemistry, the process requires the spliceosome - a multimegadalton complex considered the most intricate macromolecular machines within the cell nucleus. The spliceosome assembles in a stepwise fashion, from small nuclear ribonucleoproteins (snRNPs) and associated proteins to form distinct landmark intermediates.
Splicing does not occur in isolation; it is a cotranscriptional process that unfolds as splice sites are exposed during transcription by RNA polymerase II (RNAPII). While most spliceosome structures have been characterized in isolation using cryo-electron microscopy (cryo-EM), the connections between spliceosomes and RNAPII during cotranscriptional splicing remain unclear. To advance understanding this crosstalk, we will stall relevant complexes using antitumor compounds that inhibit splicing. We will investigate the complexes by applying an integrative approach that combines cryo-EM with biochemistry, mass spectrometry and functional analyses.
Project aims
- Reconstitution of spliceosomes
- Biochemical characterization of the stalled spliceosomes
- Characterization of the 3D structure by cryo-EM
- Analysis of the proteome and subunit proximities in endogenous spliceosomes
Further details & requirements
Overview
We aim to investigate how spliceosomes interact with RNAPII to coordinate the assembly of the spliceosome. As spliceosomes are also targets for antitumor drugs, we will reconstitute and study the structure of these spliceosomes stalled by splicing inhibitors with antitumor properties. We will first explore strategies for the in vitro reconstitution of relevant spliceosomes (1), perform a biochemical and biophysical characterization of these spliceosomes (2), and investigate the spliceosomes by cryo-EM (3). As a complementary strategy, we will purify and analyze endogenous spliceosomes from human cell nuclei (4).
1. Reconstitution of spliceosomes
We routinely employ methodologies for engineering and isolation of spliceosomes (De et al., 2015, Cretu et al., 2021) We assemble spliceosomes in vitro from nuclear extracts from HeLa cells. As RNA substrates, we will use reporter pre-mRNA molecules synthesized with T7 polymerase, fluorescently labeled, and tagged with aptamers for purification. To stall the spliceosome at a defined state, we will add the splicing inhibitors to the nuclear extract during spliceosome preparation by adapting our routine protocols (Cretu et al., 2021).
2. Biochemical and biophysical characterization of the stalled spliceosomes
We will upscale the preparation of stalled spliceosomes from large amounts of HeLa nuclear extracts by chromatography and gradient ultracentrifugation (Cretu et al., 2021, Schmitzova et al., 2023). We use a bioreactor for HeLa cell cultivation in a continuous perfusion system to produce the required amount of nuclear extracts. The composition and homogeneity of the complexes will be analyzed using electrophoresis, mass spectrometry, and mass photometry. Furthermore, we will investigate the molecular organization of the particles by protein-protein crosslinking coupled to mass spectrometry, thus estimating proximities between the spliceosome and interacting components(Cretu et al., 2016).
3. Characterization of the 3D structure by cryo-EM
To stabilize the purified spliceosomes, we will likely subject them to chemical fixation in gradient ultracentrifugation (the Grafix method) before conducting electron-microscopic investigations. Negative-staining electron microscopy will further assess the particles' homogeneity, when a preliminary 3D reconstruction will be generated at low resolution. For cryo-EM analysis, we will test various grids, including types of gold grids, which in our hands led to the best results with spliceosomal particles (Cretu et al., 2021). For data collection, we will first use the Glacios cryo-electron microscope from ICR. CryoSPARK and RELION will be used for automated particle-picking and 2D classification, as well as for all the 3D classifications and 3D refinements. For structural characterization at near-atomic resolution, we will further collect data from a Titan Krios instrument from the Crick Institute, where our lab has regular access. The GPU cluster from ICR will be extensively used for data processing. Structural insight into the role of these particles may become apparent as soon as the cryo-EM structures will be determined at sufficient resolution to compare them with available structures of spliceosomes.
4. Analysis of the proteome and subunit proximities in endogenous spliceosomes
The strategy outlined above involves assembling spliceosomes using nuclear extracts, purified complexes, and precisely defined RNA-DNA constructs. In addition, we will employ an alternative strategy focused on analyzing endogenous spliceosomes—those that naturally emerge from chromatin as RNAPII translocates through genes. This method complements the previous one, allowing to capture additional factors that may be associated with spliceosomes. Indeed, endogenous spliceosomes have already been observed as they are released from chromatin through gentle fragmentation(Nojima et al., 2018). These spliceosomes are associated to RNAPII, indicative of their cotranscriptional formation. We will use differents tartegies to purify endogenous spliceosomes, and characterize their proteome by mass spectrometry. Additionally, crosslinking combined with mass spectrometry will help estimate the proximities and potential interfaces between the subunits of the endogenous spliceosomes. The crosslinks can be used to model existing structures' proximities and extend the insight of the models obtained from point 3.
Expected outcome
This research can provide substantive insights into the interactions and three-dimensional coordination between spliceosomes and RNAPII during cotranscriptional splicing. Moreover, it will enhance our understanding of the mechanisms of splicing inhibitors that possess antitumor properties, broadening our perspective on their action in therapeutic contexts.
Note: the ICR’s standard minimum entry requirement is a relevant undergraduate Honours degree (First or 2:1).
Pre-requisite qualifications of applicants:
- BSc or Master in molecular biology or biochemistry
- Basic experience in cloning, recombinant expression, and protein purification
- Basic knowledge of structural biology
Intended learning outcomes:
- recombinant protein expression
- purification of spliceosomes
- RNA structural biology
- Single particle electron cryo-microscopy
- Protein Biochemistry
ALEXANDER, R. & BEGGS, J. D. 2010. Cross-talk in transcription, splicing and chromatin: who makes the first call? Biochem Soc Trans, 38, 1251-6.
CRETU, C., GEE, P., LIU, X., AGRAWAL, A., NGUYEN, T. V., GHOSH, A. K., COOK, A., JURICA, M., LARSEN, N. A. & PENA, V. 2021. Structural basis of intron selection by U2 snRNP in the presence of covalent inhibitors. Nat Commun, 12, 4491.
CRETU, C., SCHMITZOVA, J., PONCE-SALVATIERRA, A., DYBKOV, O., DE LAURENTIIS, E. I., SHARMA, K., WILL, C. L., URLAUB, H., LUHRMANN, R. & PENA, V. 2016. Molecular Architecture of SF3b and Structural Consequences of Its Cancer-Related Mutations. Mol Cell, 64, 307-319.
DE, I., BESSONOV, S., HOFELE, R., DOS SANTOS, K., WILL, C. L., URLAUB, H., LUHRMANN, R. & PENA, V. 2015. The RNA helicase Aquarius exhibits structural adaptations mediating its recruitment to spliceosomes. Nature structural & molecular biology, 22, 138-44.
KASTNER, B., WILL, C. L., STARK, H. & LUHRMANN, R. 2019. Structural Insights into Nuclear pre-mRNA Splicing in Higher Eukaryotes. Cold Spring Harb Perspect Biol, 11.
NOJIMA, T., REBELO, K., GOMES, T., GROSSO, A. R., PROUDFOOT, N. J. & CARMO-FONSECA, M. 2018. RNA Polymerase II Phosphorylated on CTD Serine 5 Interacts with the Spliceosome during Co-transcriptional Splicing. Mol Cell, 72, 369-379 e4.
SCHMITZOVA, J., CRETU, C., DIENEMANN, C., URLAUB, H. & PENA, V. 2023. Structural basis of catalytic activation in human splicing. Nature, 617, 842-850.
SHINE, M., GORDON, J., SCHARFEN, L., ZIGACKOVA, D., HERZEL, L. & NEUGEBAUER, K. M. 2024. Cotranscriptional gene regulation in eukaryotes and prokaryotes. Nat Rev Mol Cell Biol, 25, 534-554.
WILKINSON, M. E., CHARENTON, C. & NAGAI, K. 2020. RNA Splicing by the Spliceosome. Annu Rev Biochem, 89, 359-388.
YAN, C., WAN, R. & SHI, Y. 2019. Molecular Mechanisms of pre-mRNA Splicing through Structural Biology of the Spliceosome. Cold Spring Harb Perspect Biol, 11.