Dr Dimitra Darambara
Group Leader: Multimodality Molecular Imaging
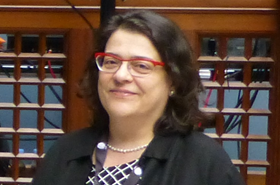
Biography
Dr Darambara’s research focuses on the development of novel imaging instrumentation for better identifying, visualising and quantifying molecular and cellular characteristics of cancer. She received her BSc (Hons) in Physics & Maths from the University of Athens, Greece. She then successfully pursued her postgraduate studies at Yale University, USA and received her PhD in Experimental Physics from the University of Surrey, UK with a full NATO research studentship (closed access).
Her PhD focused on developing a novel generic imaging detector based on Si-memory devices and an associated spatial diagnostic imaging technique for varied targets (e.g. biological cells) with biomedical, security and industrial applications. The detector that she developed was a breakthrough in detector technology and has been included in “Radiation Detection and Measurement” by G.F. Knoll, the standard reference book of the field.
Afterwards she held positions at CERN as a Research Fellow, at the University of Surrey as a NATO Research Fellow and at University College London and back at University of Surrey as a Senior Research Fellow sponsored by the Wellcome Trust with a research career development fellowship working on emerging medical imaging techniques and technologies for quantitative molecular imaging.
She is a Member of the Institute of Physics (IOP), the Institute of Physics and Engineering in Medicine (IPEM) and the Institute of Electrical and Electronic Engineers (IEEE). She is the current Chair of the IOP Medical Physics Group, the Chair of the Board of Trustees of the Mayneord-Phillips Trust and member of the Royal Academy of Engineering (RAE) Panel for Biomedical Engineering.
The detector component is the heart of every detection system, whether this is a medical imaging scanner or a huge sophisticated particle detector at CERN, and it provides a wealth of quantifiable information, whether about a cell/tissue/organ/body or a particle identity. Hence, a detector with the highest possible detection efficiency, sensitivity and spatial, spectral and temporal resolutions means an imaging system with the best achievable image quality and quantitative accuracy for early and reliable cancer diagnosis and effective treatment.
She believes that it is a real challenge to bring new technologies and ideas from the basic physics lab into the clinical practice with direct impact on the quality of cancer patients’ life, but it is also fascinating, inspirational and rewarding.
Outside of work Dimitra enjoys sailing, swimming and playing piano.